INTRODUCTION
The quality of honey can be affected by several factors, such as during harvest, water content, color, taste, and aroma of honey. Harvesting honey necessitates careful timing when it is ripe and the bees are starting to close the nectar cells. The water content of honey also affects the quality by containing approximately 17-21% water. Honey is rich in simple carbohydrates because the enzymes diastase and invertase are added when the nectar is sucked and regurgitated. The diastase enzyme is produced by bees during the ripening process and is used to assess the quality of honey (Sukmawati et al. 2015).
Based on data from Riskesdas (2018), the prevalence of diarrhea in Indonesia is 37.88% and has increased in 2019 to 40% in toddlers (RI Ministry of Health 2020). Furthermore, the age group with the highest prevalence of diarrhea was 1 to 4 years (11.5%) and infants (9%). According to the WHO (2018), gastroenteritis in the general population has a mortality rate of 525,000 deaths per year in children under 5 years with an incidence rate of 1.7 million. This condition makes gastroenteritis the second leading cause of death due to infectious diseases. Acute gastroenteritis is diarrhea that lasts up to 14 days and is characterized by an increase in stool volume, frequency, and water content. The most common causes of diarrhea are infections in the form of viruses, bacteria, and parasites. It is often caused by an infectious disease from Staphylococcus aureus and Escherichia coli bacteria, which is still a major problem in public health (Muziburrahman et al. 2022).
Staphylococcus aureus is a round gram-positive bacteria arranged such as clusters of grapes and is quite active in its division, which is commonly found in burns and hospitals (Yuliati 2017). On the other hand, escherichia coli is a gram-negative rod-shaped bacterium and can cause various diseases, such as diarrhea, which has an incubation period of 10-24 hours before showing symptoms (Pratiwi 2018). Antibacterial compounds have been widely used to treat diseases caused by bacteria. It is imperative to adopt preventive measures to hinder the escalating resistance of bacteria to drugs, as over time, these pathogenic bacteria can develop resistance to antibacterial compounds (Panjaitan et al. 2018).
One way to prevent the emergence of bacterial resistance to synthetic antibacterials is the use of natural ingredients having antibacterial activity, including honey (Hanifa et al. 2020). The production of honey occurs through the transformation of nectar, a process that involves the reduction of water content within both the bee's body and the beehive. The various types of honey that exist and their potential to be used as an antibacterial agent promote this study. Some of the compounds present in honey are proteins, flavonoids, and low pH allowing for the inhibition of bacterial growth. According to Nayik and Nanda (2015), light-colored honey contains less phenol than dark-colored.
The phytochemical content of each honey varies depending on the origin of the nectar from the honey. This content is useful as an antioxidant, antibacterial, anti-inflammatory, antiviral, and antidiabetic agent, as well as affects the human body's metabolism properly, hence, they have the potential to improve health and prevent various diseases (Liu et al. 2013). Honey is classified as monofloral (one type of plant) or multi floral (several types of plants) depending on the origin of its nectar (Soares et al. 2018; Zhang et al. 2019). Presently, monofloral honey from Apis mellifera bees from Indonesia has antibacterial compounds. The effectiveness of suppressing bacterial growth varies in relation to the content of active antibacterial compounds found in honey, as well as the extractive substances derived from nectar. A total of 9 honey specimens of the monofloral species Apis mellifera from Indonesia were characterized in this study. Therefore, this study aimed to determine the activity of the diastase enzyme and the effectiveness of monofloral honey derived from Apis mellifera bees as a natural antibacterial agent against Staphylococcus aureus and Escherichia coli.
MATERIALS AND METHODS
Materials
The materials used in this study included 9 specimens of Apis mellifera honey, distilled water, starch, iodine, NaCl, acetate buffer, Staphylococcus aureus ATCC6538 and Escherichia coli ATCC8739 bacterial cultures, Nutrient Broth (NB) and Mueller Hiton Agar (MHA) media, ciprofloxacin, and aquabides.
Sample Preparation
Samples of monofloral honey from Indonesia, as shown in Table 1, were stored in the Laboratory of the Biochemistry Master’s Study Program, Faculty of Mathematics and Natural Sciences, Bogor Agricultural University.
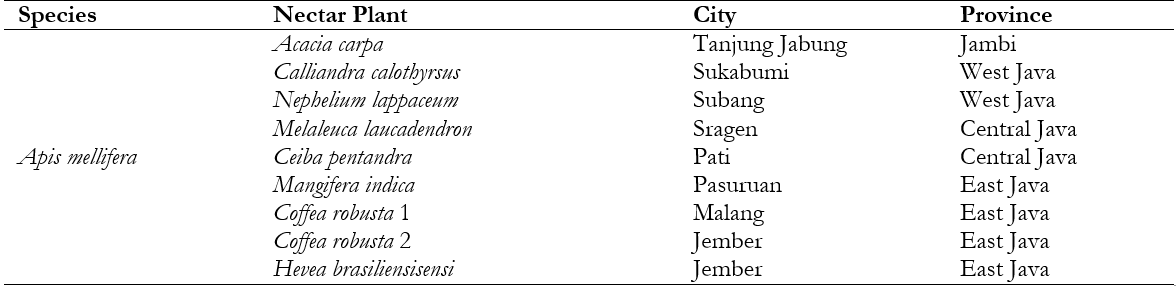
Water Content
The water content of monofloral honey was measured using a refractometer according to the Indonesian National Standard (2018). After dripping one drop of honey sample onto the prism, it was closed and pointed towards the light, and the result was read from the blue line on the white scale.
Diastase Enzyme Activity
The water content of monofloral honey was measured using a refractometer according to the Indonesian National Standard (2018). After dripping one drop of honey sample onto the prism, it was closed and pointed towards the light, and the result was read from the blue line on the white scale.
Diastase enzyme activity (DN) was calculated by the following formula:
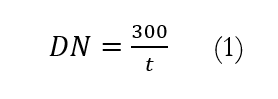
DN = diastase enzyme activity
t = time used to reach the value of A<0.235
Antibacterial Activity
The antibacterial activity of monofloral honey was analyzed according to the method of Devi (2022) with modifications. Furthermore, bacterial cultures of Staphylococcus aureus and Escherichia coli rejuvenated in 50 mL nutrient broth (NB) media were used as tested strains for antibacterial testing. Each strain was incubated for 24 hours at 37ºC and the culture that grow were subjected to the disk diffusion test. The Mueller Hiton Agar (MHA) media used in the test were sterilized using an autoclave at 121˚C for 15 minutes. Furthermore, the MHA media was then poured into a sterile Petri dish until solidified and the bacterial culture was streaked on the MHA media with a known OD value. Sterile discs were left overnight to absorb the honey sample to be tested at a concentration of 100% and then dried in an oven at 45˚C for 2 hours. The prepared discs were placed on a bacterial culture scratch on MHA and incubated at 37˚C for 24 hours. Furthermore, the clear zone formed around the disc was measured with a Vernier caliper. Ciprofloxacin at a concentration of 100 ppm (0.1%) and 25 µL of blank distilled water was used as the positive and negative control, respectively.
Data Analysis
Each experiment was carried out in triplicate, and the diastase enzyme activity and antibacterial activity resulting in each replicate were written as the mean ± standard deviation (n=3). The one-way ANOVA test was used to analyze data on all honey samples with a 0.05 level of significance (α) using SPSS version 25. A p-value < 0.05 indicated that the data were significantly different, and should be further tested. Duncan's test was used to determine the effect of significant differences in each monofloral honey. Furthermore, the classification of monofloral honey based on diastase enzyme and antibacterial activity was determined by the principal component analysis (PCA) chemometric method with Minitab 16.
RESULTS AND DISCUSSION
Water Content
Among the 9 samples analyzed, the water content of honey adhered to predetermined standards. Coffea robusta 2 honey had the lowest water content at 17.50%, while Calliandra calothyrsus had the maximum at 22.00% determined by SNI. The moisture content of Nephelium lappaceum, Melaleuca laucadendron, Ceiba pentandra, Mangifera indica, Coffea robusta 1, Acacia carpa, and Hevea brasiliensisensi were 21.50%, 20.50%, 20.50%, 19.50%, 19.00%, 18.50%, and 18.00%, respectively. The ANOVA statistical analysis supported this result, showing a significant difference in the water content of the 9 monofloral honeys (p-value < 0.05). This showed that differences in plants as a source of nectar had an impact on the water content of honey. The results of Duncan's test (α=0.05) on the water content of 9 monofloral honeys showed 8 groups, namely (1) Calliandra calothyrsus, (2) Nephelium lappaceum L., (3) Melaleuca laucadendron L. and Ceiba pentandra (L.) Gaertn, (4) Mangifera indica, (5) Coffea robusta 1, (6) Acacia carpa, (7) Hevea brasiliensisensi M., and (8) Coffea robusta 2. These groups were categorized based on the proximity of their water content, as shown in Figure 1.
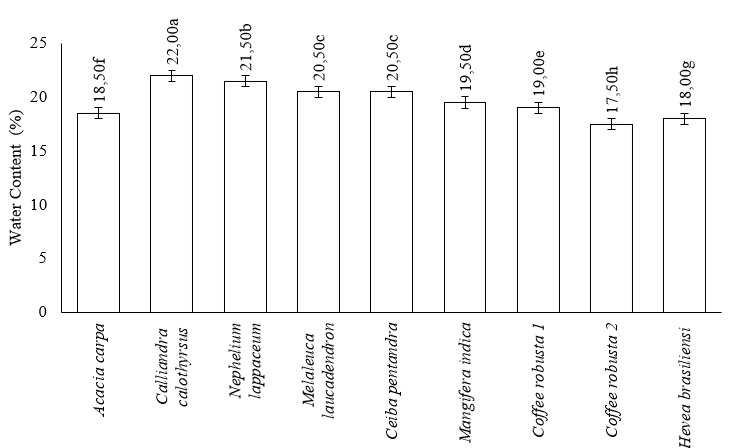
Note: Different lowercase letters show statistically significant differences (α=0.05).
The water content in honey determines the stability of fermentation because it allows easy fermentation over time. Therefore, honey needs to be subjected to heat treatment to prevent fermentation by sugar-tolerant yeasts and maintain its liquid state (Singh and Singh 2018). Relative humidity (Rh) in Indonesia ranges from 60–90%, indicating that the water content in honey is approximately 18.3–33.1%. Honey is hygroscopic, and must be stored in a place that is not permeable to air. According to Pujiarti et al. (2021), signs of a fermentation process are the foam and gas produced by honey. Hasan et al. (2020) reported that the antibacterial activity of multiflora honey from Riau RHU4 has the lowest water content of 16.45% and RHI2 honey of 20.56%.
The fermentation process in yeast can be significantly influenced by the high water content in honey, leading to a decline in its overall quality. Moreover, the shelf life of honey is affected by its water content and a higher water content results in a relatively short shelf life. According to Hasan et al. (2020), honey can absorb up to 33% water by weight in moist air. Good-quality honey has a low water content, indicating that thicker honey has a lower water content (Swari et al. 2019). Honey harvested at an older age contains less water than at a younger age. Generally, at harvest ages < 14 days, the water content of honey is relatively high. According to Nasharuddin et al. (2022), Indonesian honey is often fermented due to its high water content of >26%. The Indonesian National Standard (2018) reported that the quality requirement of water content in honey was a maximum of 22%. A previous study showed a correlation between the water and sugar content of honey, and the honey crystallization process was natural (Nasharuddin et al. 2022).
Diastase Enzyme Activity
The average results of diastase enzyme activity in monofloral honey were shown in Figure 2. The highest diastase enzyme activity in Mangifera indica, Nephelium lappaceum, and Coffea robusta 2 was 20.00 DN, while the lowest was in Calliandra calothyrsus at 4.00 DN. Furthermore, the diastase enzyme activity of Melaleuca laucadendron, Ceiba pentandra, and Hevea brasiliensisensi was 10.00 DN, whereas Acacia carpa and Coffea robusta 1 were 6.67 DN and 5.00 DN, respectively.
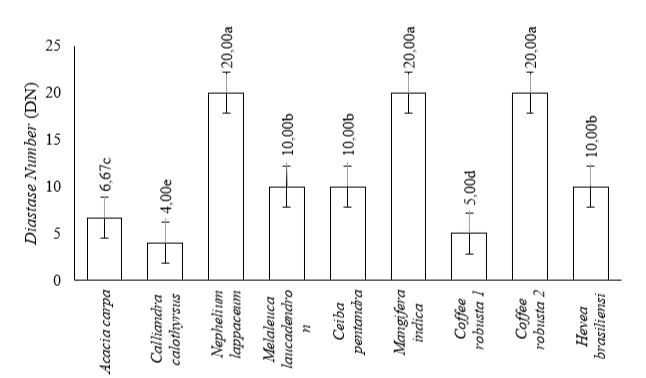
Note: Different lowercase letters show statistically significant differences (α=0.05)/.
These results were supported by ANOVA statistical analysis which showed a significant difference between the 9 monofloral honey (p-value < 0.05) based on their diastase enzyme activity. This showed that differences in plants as a source of nectar affected it. It also showed the impact of the differences in plants as a source of nectar. The results of Duncan's test (α=0.05) on the activity of the diastase enzyme from 9 monofloral honey showed 5 groups, namely (1) Mangifera indica, Nephelium lappaceum L., and Coffea robusta 2, (2) Melaleuca laucadendron L., Ceiba pentandra (L.) Gaertn, and Hevea brasiliensisensi M., (3) Acacia carpa, (4) Coffea robusta 1, and (5) Calliandra calothyrsus M. These monofloral honey awerere categorized based on their proximity to the activity of the diastase enzyme they possess, as shown in Figure 2.
The diastase enzyme is one of many different types found in every variety of honey and contributes to its nutritional value. Bees introduce the diastase enzyme during the ripening of the honey. This enzyme breaks the glucosidic bonds in starch and other oligo- and polysaccharides into simple sugars. According to Singh and Singh (2018), adequate heating and storage are crucial to retaining the market value of honey since the diastase enzyme is extremely sensitive to heat.
According to Ichsan et al. (2022), in the chemical test with the diastase enzyme, 17 out of 22 samples were found to have good quality because they met the SNI requirement. The Diastase Number (DN) value ranged from 0.64 to 23.11, while for fake honey, the value of DN was 0, indicating that it was undetectable. The absence of the diastase enzyme in a honey sample can serve as an indicator of potential adulteration, suggesting that the sample may be classified as fake honey. Such samples solely consist of sugars without the presence of the diastase enzyme. Furthermore, Tulandi (2019) showed that storage temperature greatly affected the quality of honey. There was a significant difference in diastase enzyme activity in each treatment at storage temperature. The best storage temperature for honey was 26˚C (room temperature) compared to hot (50˚C) and cold (±5˚C) temperatures.
The activity of this enzyme decreased with storage and heating time, as indicated by its measurement through DN. It is observed that diastase activity decreases as the temperature rises, and the decline becomes more significant with higher temperature increments. The diastase activity decreases quickly during isothermal heating at a constant temperature but increases once again as the number of heating cycles increases (Singh and Singh 2018). According to Harjo et al. (2015), diastase activity in rubber and rambutan honey was 11.89 DN and 11.58 DN, respectively. The presence of the diastase enzyme in honey can be attributed to the bee's ability to produce saliva. Consequently, a higher value of the diastase enzyme indicates a greater concentration of bee saliva contained in honey.
A decrease in the value of the diastase enzyme activity can occur in the storage process before testing. Therefore, it is advisable to conduct diastase enzyme testing shortly after the honey is harvested to ensure accurate assessment. The activity of the diastase enzyme is an indicator of the quality of honey because it can indicate its processing and storage time. According to Pujiarti et al. (2021), storage can significantly affect the inactivation of enzymes. Honey products usually do not meet requirements for commercialization after they have been heated, reheated, or stored for a long time, because the diastase activity is below the quality requirements. According to the Indonesian National Standard (2018), the quality requirements for the value of honey diastase activity are more than 3 DN.
Antibacterial Activity
The smallest clear zone for Staphylococcus aureus was 14.73 mm in Mangifera indica, while the largest was 19.47 mm in Coffea robusta 2. The clear zones for Acacia carpa, Melaleuca laucadendron, Coffea robusta 1, Ceiba pentandra, Hevea brasiliensisensi, Nephelium lappaceum, and Calliandra calothyrsus were 18.53 mm, 17.73 mm, 17.03 mm, 16.12 mm, 16.10 mm, 16.03 mm, and 15.73 mm, respectively, as shown in Figure 3. The smallest clear zone for Escherichia coli was 21.53 mm in Nephelium lappaceum, while the largest was 27.93 mm in Ceiba pentandra. The values for Coffea robusta 2, Acacia carpa, Coffea robusta 1, Melaleuca laucadendron, Mangifera indica, Hevea brasiliensisensi, and Calliandra calothyrsus were 26.13 mm, 24.60 mm, 24.53 mm, 22.53 mm, 22.07 mm, 21.90 mm, and 21.60 mm, respectively, as shown in Figure 4.
The positive control on both bacteria showed that a clear zone was formed. This is consistent with the ANOVA statistical analysis, showing that the 9 monofloral honey were significantly different (p-value < 0.05) based on their antibacterial activity. The results of Duncan's test (α=0.05) on the inhibition zone of Staphylococcus aureus bacteria from 9 monofloral honey showed 6 groups, namely (1) Coffea robusta 2 and Acacia carpa, (2) Acacia carpa and Melaleuca laucadendron L., (3) Melaleuca laucadendron L. and Coffea robusta 1, (4) Coffe robusta 1, Ceiba pentandra (L.) Gaertn, and Nephelium lappaceum L., (5) Ceiba pentandra (L.) Gaertn, Hevea brasiliensisensi M., Nephelium lappaceum L., and Calliandra calothyrsus M., and (6) Mangifera indica. These monofloral honey were grouped based on the proximity of their inhibition zones, as shown in Figure 3. Furthermore, Duncan's test at α=0.05 on the inhibition zone of Escherichia coli bacteria showed that 4 groups, namely (1) Ceiba pentandra (L.) Gaertn, (2) Coffe robusta 1, (3) Acacia carpa and Coffea robusta 2, and (4) Melaleuca laucadendron L., Mangifera indica, Hevea brasiliensisensi M., Calliandra calothyrsus M., and Nephelium lappaceum L. They were groups based on the proximity of their inhibition zones, as shown in Figure 4.
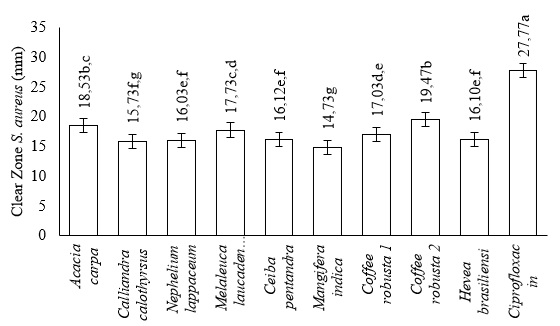
Note : Different lowercase letters show statistically significant differences (α=0.05).
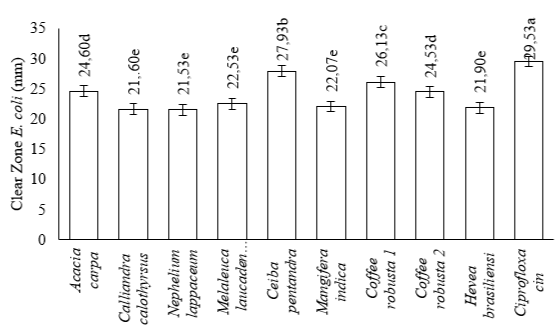
Note: Different lowercase letters show statistically significant differences (α=0.05).
The clear zone value is determined by subtracting the diameter of the disc used from the measured clear zone diameter. The effectiveness of bacterial inhibition by honey is influenced not only by physicochemical characteristics but also by its bioactive compounds. Furthermore, the content of bioactive compounds is obtained from nectar sources that are taken and processed by honey bees (Hasan et al. 2020).
The monofloral honey antibacterial test was carried out on two test bacteria, namely Staphylococcus aureus (Gram-positive) and Escherichia coli (Gram-negative). Furthermore, antibacterial activity was determined based on the diameter of the clear zone formed after incubation. This test used the antibiotic ciprofloxacin (100 ppm) as the positive control. According to the Clinical Laboratory Standard Institute (CLSI) (2013), antibacterial activity is classified as weak or lacking when the resulting inhibition zone is ≤12 mm. Similarly, it is classified as medium and strong when the inhibition zone is 13-17 mm and ≥18mm, respectively. Based on this classification, the test results showed that monofloral honey had moderate to strong antibacterial activity against Staphylococcus aureus. This was because the diameter of the resulting inhibition zone was in the range of 14.73 mm and 19.47 mm. The antibacterial activity of monofloral honey against Escherichia coli was classified as strong because the diameter of the resulting inhibition zone was greater than 18 mm, ranging from 21.53 mm to 27.93 mm.
According to Hasan et al. (2020), the smallest inhibition zone of Staphylococcus aureus for multi-floral honey from Riau was 7.84 mm for 20% RHI1 honey, while the largest for RHI2 80% honey was 19.25 mm. The smallest inhibition zone of Escherichia coli in RHI1 honey with a concentration of 40% was 1.22 mm, while the largest inhibition zone in PLW1 60% honey was 4.58 mm. According to Kaligis et al. (2020), the minimum inhibitory concentration (MIC) of forest honey toward Staphylococcus aureus, Escherichia coli, and Pseudomonas aeruginosa is 12.5%, 12.5%, and 25%, respectively. In this study, there was no minimum bactericidal concentration (MBC) in either forest or black honey. Furthermore, the antibacterial effect of forest honey is superior to that of black honey by a small margin.
Gram-negative bacteria tend to be more sensitive to the antibacterial substances contained in honey than Gram-positive. They also have hydrophilic groups that easily bind to polar compounds, making the antibacterial activity of honey more effective against Gram-negative bacteria than Gram-positive (Wadi 2022). Furthermore, the study conducted with monofloral honey from Indonesia showed that Escherichia coli was the most influential bacteria. This can be attributed to osmosis, facilitated by the high sugar content of honey, causing water to flow out of the cells, such that the resulting pure and undiluted honey prevents the growth of germs. According to Almasaudi (2021), Dehydration also causes cells to shrink, which hinders their ability to survive in a hypertonic sugar solution, thereby reducing the antibacterial potential.
The vast majority of microbes normally grow in neutral environments with pH values between 6.5 and 7.5. One of the most distinguishing characteristics of the antibacterial qualities of honey is its acidity, which ranges between pH 3.2 and 4.5. Its acidity is caused by the presence of a significant organic acid (gluconic) that is present in the sample at a concentration of 0.5% (w/v). The natural glucose oxidase enzyme converts glucose into glycogen acid, which is a particularly effective antibacterial agent. Furthermore, low pH in pure and undisturbed honey can add to its antibacterial capabilities, when diluted in food or other body fluids (Almasaudi 2021).
Cianciosi et al. (2018) reported that flavonoids and phenolic acids were among the broad group of compounds known as polyphenols and distinguished by their phenolic structures. These biological components are converted from nectar to honey and play a significant role in the health benefits of honey. The presence of numerous phenolic chemicals in honey provides a plausible explanation for its antibacterial properties (Gunes et al. 2017).
Several studies explored the antibacterial activity of phenolic acids in honey. Among these acids, caffeic was found to induce oxidative stress and chlorogenic acid increases membrane permeability, resulting in cytoplasmic and nucleotide leakage (Gorniak et al. 2019). Furthermore, gallic acid induces intracellular leakage as a result of the breakdown of cell membranes and increased hole formation (Shi et al. 2016). P-coumaric acid disrupts cell membranes and bacterial DNA brushing, and syringic acid causes cell membrane dysfunction (Borges et al. 2013). The antibacterial mechanisms of flavonoids found in honey include apigenin and kaemferol, which can inhibit DNA gyrase. Galanin inhibits peptidoglycan synthesis from ribosomes and luteolin inhibits FAS-I in mycobacteria and inhibits DNA helicase DnaB and RecBCD. On the other hand, myricetin inhibits DNA B helicase, pinocembrin induces cell lysis (Collins et al. 2019), and catechins contribute to the generation of H2O2 (Das et al. 2015).
Honey Clusterization
The results of the score plot on the antibacterial activity of Staphylococcus aureus and Escherichia coli and diastase activity show 4 main clusters of monofloral honey from Indonesia with different nectar sources (Figure 5). The first cluster (Figure 5a) consists of Hevea brasiliensisensi from Jember. The second cluster (Figure 5b) consists of Mangifera indica from Pasuruan and Nephelium lappaceum from Subang. The third cluster (Figure 5c) consists of Acacia carpa from Tanjung Jabung and Calliandra calothyrsus from Sukabumi. Finally, the fourth cluster (Figure 5d) consists of Melaleuca laucadendron honey from Sragen, Ceiba pentandra from Pati, Coffea robusta 1 from Malang, and Coffea robusta 2 from Jember.
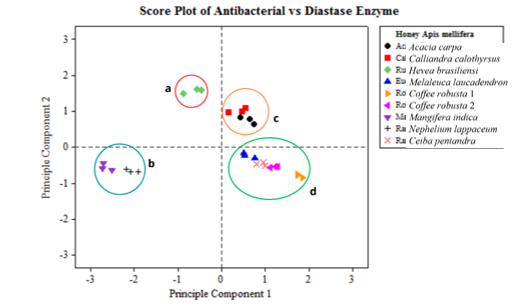
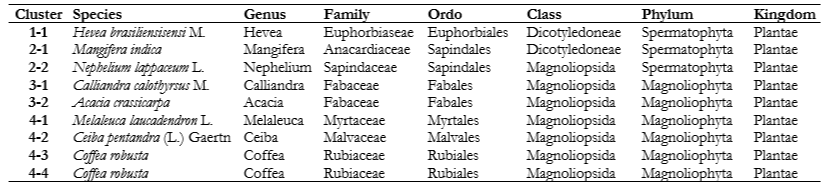
The clustering of monofloral honey derived from 9 nectar-sourced plants in Indonesia was determined using the multivariate PCA method. This analysis considered both the antibacterial activity and diastase enzymes. The results showed that there are groups with similarities and some exhibit dissimilarities. The PCA method is used to simplify data and enhance readability by transforming the data linearly to form a new coordinate with maximum variance (Ghani et al. 2016).
The arrangement of taxonomy is based on phylogenetic and chemotaxonomy. In this context, plants that share a close kinship tend to possess similar types and homologies of secondary metabolite content. The identified chemical compounds have antibacterial activity. For example, cluster 1 is the genus Hevea, which contains flavonoids (Evahelda et al. 2017). Cluster 2 is of the Sapindales order, and it contains acetophenone, butanoic acid, and benzyl alcohol (Rohman 2017). Cluster 3 belongs to the Fabaceae family, containing protocatechuic, gallic, caffeic, and ascorbic acid (Obisitioiu et al. 2021). Cluster 4 belongs to the Magnoliopsida class, which is characterized by aromatic compounds, such as sesquiterpenes, isoflavonoids, and benzylisoquinone alkaloids (Chassagne et al. 2021).
The differences in honey characteristics, such as taste, aroma, color, and composition, can be attributed to the diverse range of plants whose nectar is collected by bees for honey production. These variations subsequently influence the antibacterial activity of honey. Furthermore, several factors contribute to the antibacterial activity of honey, namely the types of flowers that take their nectar from different areas and their high sugar content (Arumsari et al. 2019).
CONCLUSION
In conclusion, the resulting diastase activity in monofloral honey includes Mangifera indica, Nephelium lappaceum, Coffea robusta 2 at 20.00 DN, Nephelium lappaceum, Ceiba pentandra, and Hevea brasiliensisensi at 10.00 DN, Acacia carpa at 6.67 DN, Coffea robusta 1 at 5.00 DN, and Calliandra calothyrsus 4.00 DN. The inhibition zones for Staphylococcus aureus on Coffea robusta 2, Acacia carpa, Nephelium lappaceum, Coffea robusta 1, Ceiba pentandra, Hevea brasiliensisensi, Nephelium lappaceum, Calliandra calothyrsus, and Mangifera indica were 19.47, 18.53, 17.73; 17.03, 16.12, 16.10, 16.03, 15.73, and 14.73 mm, respectively. Furthermore, the inhibition zones for Escherichia coli on Ceiba pentandra, Coffea robusta 2, Acacia carpa, Coffea robusta 1, Melaleuca laucadendron, Mangifera indica, Hevea brasiliensisensi, Calliandra calothyrsus, and Nephelium lappaceum were 27.93, 26.13, 24.60, 24.53, 24.53, 24.07, 21.90, 21.60, and 21.53 mm, respectively. In this study, clusterization analysis was based on the antibacterial and diastase activity, namely cluster 1 consisting of Hevea brasiliensisensi, cluster 2 comprising Mangifera indica and Nephelium lappaceum, cluster 3 containing Acacia carpa and Calliandra calothyrsus, as well as cluster 4 involving Melaleuca laucadendron, Ceiba pentandra, Coffea robusta 1, and Coffea robusta 2. Therefore, it is necessary to carry out antibacterial testing of other bacteria, specifically Salmonella typhi, and determine MIC of honey with the best antibacterial activity in various concentration variations.
ACKNOWLEDGMENTS
The authors are grateful to the Department of Biochemistry, IPB University, for the support provided during this study.
AUTHORS’ CONTRIBUTION
Rara Annisaur Rosyidah designed and conducted all the experiments, analyzed the data, performed the data visualization, and wrote the manuscript. Akhmad Endang Zainal Hasan and Dimas Andrianto assisted in conducting the experiments, performed the data visualization, and wrote the manuscript. All authors have read and approved the final manuscript.
CONFLICT OF INTEREST
The authors declare that there are no competing interests. This current study does not involve experiments on animals or human subjects and the data are available from the authors.
REFERENCES
- Almasaudi S. 2021. The antibacterial activity of honey. Saudi J Biol Sci 28(2021): 2188-2196.
- Andayani RP. 2020. Honey as a complementary therapy to overcome diarrhea in children under five. Perintis’s Health J 7(1): 64-68.
- Arumsari A, Herawati D, Afrizal M. 2019. Antibacterial activity test of several types of honey against Pseudomonas aeruginosa and Staphylococcus aureus by agar diffusion method. JIF Framasyifa 2(1): 26-32.
- Borgen A, Ferreira C, Saavedra MJ, Simoes M. 2013. Antibacterial activity and mode of action of ferulic and gallic acid against pathogenic bacteria. Microb Drug Resist 19(4): 256-265.
- Chassagne F, Samarakoon T, Porras G, Lylees JT, Dettweuler M, Maequez L, Salam AM, Habih S, Farrokhi DR, Quave CL. 2021. A systematic review of plant with antibacterial activities: a taxonomic and phylogenetic perspective. Front Pharmacol 11(2021): 1-29.
- [CLSI] Clinical Laboratory Standart Institute. 2013. Performance Standard for Antimicrobial Susceptibility Testing. USA(US): Twentieth Information Supplement.
- Collins W, Lowen N, Blake DJ. 2019. Caffeic acid esters are effective bactericidal compounds. Biomolecules 9(8): 1-13.
- Das A, Datta S, Mukhrejee S, Bose S, Ghosh S, Dhar P. 2015. Evaluation of antioxidative, antibacterial, and probiotic growth stimulatory activities of Sesamum indicum honey containing phenolic compounds and lignin. LWT-Food Sci Tech 61(1): 244-250.
- Evahelda E, Pratama F, Malahayati N, Santoso. 2017. Physical and chemical properties of honey from rubber tree nectar in Central Bangka District, Indonesia. Agritech 37(4): 363-368.
- Ghani ZDFA, Husin JM, Rashid AHA, Shaari K, Chik Z. 2016. Biochemical studies of Piper betle L extract on the obese treated animal using 1H-NMR-based metabolomic approach of blood serum samples. J Ethnopharmacol. 194(2016): 1-25.
- Gonzalez-Ceballos L, Fernandez-Muino MA, Oses SM, Sancho MT, Ibeas S, Ruiz JAR, Vallejos S. 2021. Polymer film as a starch azure container for the easy diastase activity determination in honey. Food Chem 335(2021): 1-5.
- Gorniak I, Bartoszewski R, Kroliczewski J. 2019. A comprehensive review of antimicrobial activities of plant flavonoids. Phytocem Rev 18(1): 241-272.
- Gunes N, Aydin L, Beleni D, Hranitz JM, Mengilig S, Selova S (2017) Stress responses of honey bees to organic acid and essential oil treatment against varroa mites. J of Agricultural Res 56(2): 175-181.
- Hanifa F, Purwaningrum R, Mustofa F, Zulfan. 2020. Effectiveness of pure honey and propolis against milk contaminating bacteria that cause foodborne disease in packaged milk. J Health 9(1): 47-52.
- Harjo SST, Radiati LE, Rosyidi D. 2015. Comparison of rubber honey and rambutan honey based on water content, diastase enzyme activity, and hydroxymethylfurfural (HMF). J Production Sci Tech Livestock Products 10(1): 18-21.
- Hasan AEZ, Herawati H, Purnomo, Amalia L. 2020. Physicochemistry of multiflora honey from Riau and its potential as an antibacterial Escherichia coli and Staphylococcus aureus. Chem. Prog 13(2): 81-90.
- Ichsan DS, Nurdin I, Hafidzah TS, Putri BS, Aurene SV. 2022. Fake honey detection and honey quality with diastase enzyme test. Poltekita: J Health Sci 16(3): 278-283.
- Kaligis CJ, Nangoy E, Mambo CD. 2020. Test the antibacterial effect of forest honey and black honey against Staphylococcus aureus, Escherichia coli, and Pseudomonas aeruginosa bacteria. eBiomedik 8(1): 112-119.
- [Ministry of Health of the Republic of Indonesia] Ministry of Health of the Republic of Indonesia. 2020. Indonesian Health Profile. Jakarta(ID): Directorate General of Disease Control and Environmental Health.
- Muziburrahman M, Husada D, Utomo B. 2022. Identification of bacteria causing diarrhea in under-five children using culture method in Bima, Indonesia. Periodic Epidemiol J 10(1): 95-102.
- Liu JR, Ye YL, Lin TY, Wang YW, Peng CC. 2013. Effect of floral sources on the antioxidant, antimicrobial, and anti-inflammatory activities of honey in Taiwan. Food Chem 139(1-4): 938-943.
- Molan P, Rhodes T. 2015. Honey: a biologic wound dressing. J Wounds 27(6): 141-150.
- Nasharuddin MA, Sunaryo, Puspitarini OR. 2022. Analysis of the quality of acacia honey, rubber, and randu produced by PT Kembang Joyo Sriwijaya. Dinamika Rekasatwa J 5(2): 169-173.
- Nayik GA, Nanda V. 2015. Physico-chemical, enzymatic, mineral, and colour characterization of three different varieties of honey from khasmir valley of India with a multivariate approach. J Food Nutr Sci 65(2): 101-108.
- Obistioui D, Covan I, Tirzui E, Herman V, Megrea M, Cucerzan A, Naescu AG, Cizma AL, Nichita I, Hulea A, Radoluv I, Alexa E. 2021. Phytochemical profile and microbiological activity of some plants belonging to the fabaceae family. Antibiotic 10(62): 1-20.
- Panjaitan R, Darmawati S, Prastiyanto M. 2018. Antibacterial activity of honey against multi-drug resistant Salmonella typhi and methicillin-resistant Staphylococcus aureus. Unimus FMIPA Education National Seminar on Science and Technology 2018. Muhammadiyah University of Semarang, Semarang [Indonesia].
- Rohman A. 2017. Physico-chemical properties and biological activities of rambutan (Naphelium lappaceum L) fruit. Res J Phytochem 11(2): 66-73.
- Shi C, Zhang X, Sun Y, Wang M, Song Y, Zheng Z, Chen Y, Liu X, Jia Z, Dong R, Cui LU, Xia X. 2016. Antimicrobial activity of ferulic acid against Cronobakter sakazakii and possible mechanism of action. Foodborne Pathog Dis 13(4): 196-204.
- Singh I, Singh S. 2018. Honey moisture reduction and quality. J Food Sci Technol 55(10): 3861-3871.
- Soares S, Grazina L, Mafra I, Costa J, Pinto MA, Duc HP, Oliveira MBPP, Amaral JS. 2018. Novel diagnostic tools for Asian (Apis cerana) and European (Apis mellifera) honey authentication. Food Res Int 105: 686-693.
- [SNI] Indonesian National Standard 8664:2018 (2018) Standardization of Honey Quality. Jakarta(ID): National Standardization Body.
- Sukmawati, Noor A, Firdaus. 2015. Analysis of the quality of Mallawa honey based on physicochemical parameters. Ind J Chem Res 2015(3): 259-262.
- Swari NKI, Wirawan R, Qomariyah N, Al Hadi K (2019) Analysis of the water content in honey using a combination of capacitance and refractive index methods. KONSTAN 4(1): 1-10.
- Tulandi SM. 2019. The effect of storage temperature on the quality of honey. SANITAS 10(1): 59-71.
- Wadi MA. 2022. In vitro antibacterial activity of different honey samples against clinical isolates. Biomes Res Int 2022: 1-8.
- World Health Organization (WHO) [Internet]. 2018. Diarrhoeal Disease. [updated 2017 May 2; cited 2023 Jan 22]. Available from: https:// www.who.int/en/news-room/fact-sheets/detail/ diarrhoeal-disease.
- Yuliati. 2017. Test the effectiveness of honey solution as an antibacterial agent in the growth of Staphylococcus aureus and Pseudomonas aeruginosae using the disk diffusion method. Med Professional J 11(1): 7-15.
- Zhang YZ, Chen YF, Wu YQ, Si JJ, Zhang CP, Zheng HQ, Hu FL. 2019. Discrimination of the entomological origin of honey according to the secretions of the bee (Apis cerana or Apis mellifera). Food Res Int 116: 362-369.